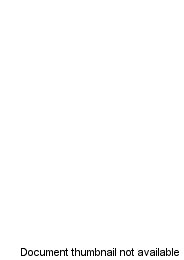
Sustainable concrete. The environmental, social and economic sustainability credentials of concrete
Publication Year
2007
Document Status
Withdrawn
Abstract
Promotes concrete as a sustainable material from its manufacture through to all stages of a building’s life. Also considers concrete’s contribution to the UK’s economy and society.
Document History
Ref: TCC/05/03.
Publisher Information
The Concrete Centre
The Concrete Centre is the central development organisation for the UK concrete sector and provides material, design and construction guidance. Their aim is to enable all those involved in the design, use and performance of concrete to realise the potential of the material. Funded by 15 major cement and concrete organisations, the Concrete Centre works alongside the British Cement Association, The Concrete Society, and the ready mix and precast concrete industries to ensure an integrated approach from the concrete sector to technical support, research, education, training and information services. The Reinforced Concrete Council and the Ready Mixed Concrete Bureau are now part of The Concrete Centre.
1st Floor
297 Euston Road
London
TCC
UK
Website: www.concretecentre.com
Email: info@concretecentre.com
Tel: 020 7963 8000